Symbiodinium
Todd LaJeunesse, John E. Parkinson, and Robert K. TrenchIntroduction
The genus Symbiodinium encompasses the largest and most prevalent group of endosymbiotic dinoflagellates known to science. These unicellular algae commonly reside in the endoderm of tropical cnidarians such as corals, anemones, and jellyfish, where they translocate products of photosynthesis to the host and in turn receive inorganic nutrients (e.g. CO2, NH4+) (Fig. 1). They are also harbored by various species of sponges, flatworms, mollusks (e.g. giant clams), foraminifera (soritids), and some ciliates. Generally, these dinoflagellates enter the host cell through phagocytosis, persist as intracellular symbionts, reproduce, and disperse to the environment (note that in most mollusks they are inter- rather than intra-cellular). Cnidarians that are associated with Symbiodinium occur mostly in warm oligotrophic (nutrient-poor) marine environments where they are often the dominant constituents of benthic communities. These dinoflagellates are therefore among the most abundant eukaryotic microbes found in coral reef ecosystems.
Symbiodinium are colloquially called "zooxanthellae" (or "zoox"), and animals symbiotic with algae in this genus are said to be "zooxanthellate". The term was loosely used to refer to any golden-brown endosymbionts, including diatoms and other dinoflagellates. Continued use of the term in the scientific literature should be discouraged because of the confusion caused by overly generalizing taxonomically diverse symbiotic relationships (Blank & Trench 1986).

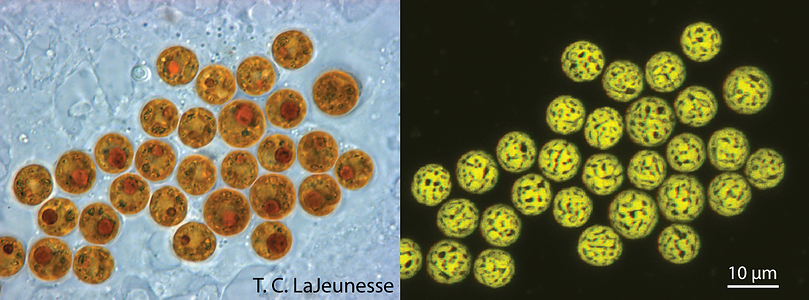
Figure 1. Light and confocal images of Symbiodinium cells in hospite (living in a host cell) within scyphistomae of the jellyfish Cassiopea xamachana. This animal requires infection by these algae to complete its life cycle. The chloroplast imaged in 3-D is highly reticulated and distributed around the cell’s periphery (photos © Todd LaJeunesse).
Characteristics
Morphological description of the genus Symbiodinium
The morphological description of the genus Symbiodinium is originally based on the type species (holotype) Symbiodinium microadriaticum (Freudenthal, 1962; see Trench & Blank, 1987). Because these dinoflagellates possess two major stages in their life history (Fig. 2), namely the mastigote (motile) and coccoid (non-motile) stages, the morphology of both are described in order to provide a complete diagnosis of the organism.

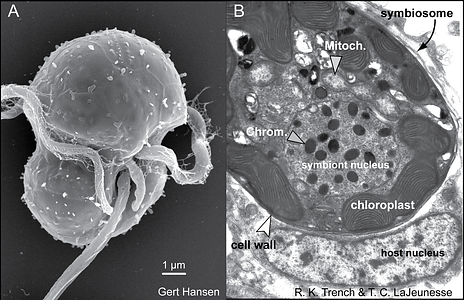
Figure 2. The life stages of dinoflagellates in the genus Symbiodinium. (A) Electron micrographs of a Symbiodinium mastigote (motile cell) with characteristic gymnodinioid morphology (S. natans; Hansen & Daugbjerg 2009) and (B) the coccoid cell in hospite. As free-living cells the mastigote allows for short-range dispersal and can exhibit chemo-taxis toward sources of nitrogen. Once within the host, these symbionts rapidly proliferate in and in many cases dominate the cytoplasm of the host cell. © Todd LaJeunesse
The mastigote cell
The mastigote, or motile cell, is gymnodinioid and athecate in appearance (Fig. 2A; Taylor 1987). The relative dimensions of the epicone and hypocone differ among species (Trench & Blank 1987). The alveoli are most visible in the motile phase but lack fibrous cellulosic structures found in thecate (“armored”) dinoflagellates. Between the points of origin of the two flagella is an extendable structure of unknown function called the peduncle (Fig. 2A). In other dinoflagellates, an analogous structure has been implicated in heterotrophic feeding and sexual recombination. In Symbiodinium, it has been suggested that the peduncle may be involved in substrate attachment, explaining why certain cells seem to spin in place (Freudenthal 1962). Compared to other gymnodinioid genera, there is little or no displacement at the sulcus (longitudinal groove) where the ends of the cingulum (transverse groove) converge.
The internal organelles of the mastigote are essentially the same as described in the coccoid cell (see below). The transition from mastigote to coccoid stage in Symbiodinium occurs rapidly, but details about cellular changes are unknown. Mucocysts (an ejectile organelle, Dodge & Greuet 1987) located beneath the plasmalemma are found in S. pilosum (Fig. 3A) and their function is unknown.

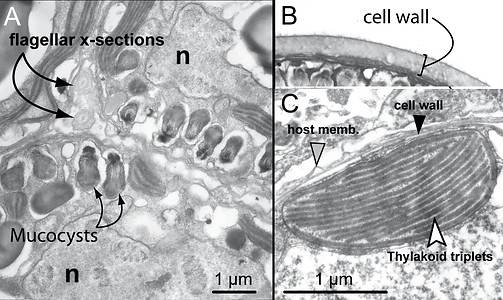
Figure 3. Fine scale features and organelles found among Symbiodinium. (A) Close-up of divided cells of a tetrad from a culture of “S. corculorum” that is genetically similar to S. pilosum (both are type A2). This particular lineage is unusual among described Symbiodinium by possessing mucocysts, and chloroplasts with parallel and peripheral thylakoid arrangements. Flagella are seen in cross section in the space between daughter cells. (B) A thick cellulosic cell wall is observed for many cultured isolates. (C) TEM of a cross-section through a chloroplast lobe at the periphery of the cell with parallel and peripheral thylakoids grouped in sets of three. © Todd LaJeunesse
The coccoid cell
The coccoid cell of Symbiodinium is spherical and ranges in average diameter from 6 to 13 µm, depending on the species (Fig. 1; Blank et al. 1989). This stage is often wrongly interpreted as a cyst; hence, in the literature the alga in hospite is often referred to as a vegetative cyst (Freudenthal 1962). The term cyst usually refers to a dormant, metabolically quiescent stage in the life history of other dinoflagellates, initiated by several factors, including nutrient availability, temperature, and day length (Lee 2008). Such cysts permit extended resistance to unfavourable environmental conditions. Coccoid Symbiodinium cells are metabolically active, as they photosynthesize, undergo mitosis, and actively synthesize proteins and nucleic acids. While most dinoflagellates undergo mitosis as a mastigote, in Symbiodinium, mitosis occurs exclusively in the coccoid cell (Fig. 4; Fitt & Trench 1983).
The cell wall:
The coccoid cell is surrounded by a cellulosic, usually smooth cell wall that contains large molecular weight proteins and glycoproteins (Trench & Blank 1987; Markell et al. 1992). Cell walls grow thicker in culture than in hospite (Fig 3B; Colley and Trench 1983). The cell membrane (plasmalemma) is located beneath the cell wall yet little is known about its composition and function in terms of the regulation of trans-membrane transport of metabolites. During karyokinesis and cytokinesis, the cell wall remains intact until the mastigotes escape the mother cell. In culture, the discarded walls accumulate at the bottom of the culture vessel. It is not known what becomes of the walls from divided cells in hospite (but see Wakefield et al. 2000). One species, S. pilosum, possesses tufts of hair-like projections from the cell wall; this is the only known surface characteristic used to diagnose a species in the genus.
The chloroplast:
Most described species possess a single, peripheral, reticulated chloroplast bounded by three membranes. The volume of the cell occupied by the chloroplast varies among species (Fig. 5; Trench & Blank 1987). The lamellae comprise three closely appressed (stacked) thylakoids, and are attached by two stalks to the pyrenoid (Trench and Blank, 1987) surrounded by a starch sheath (Fig. 4). In three of the described species, the thylakoids are in parallel arrays, but in S. pilosum, there are also peripheral lamellae. There are no thylakoid membranes invading the pyrenoid, which is unlike other symbiotic dinoflagellates (see Trench & Winsor 1987; Banaszak et al. 1993). The lipid components of thylakoids include the galactolipids (monogalactosyl-diglycerides, digalactosyl-diglycerides), the sulpholipid, (sulphoquinovosyl-diglyceride), phosphatidyl glycerol, and phosphatidyl choline. Associated with these are various fatty acids (Diaz-Almeyda et al. 2010). The light-harvesting and reaction centre components in the thylakoid membrane include a water-soluble peridinin-chlorophyll (chl.) a-protein complex (PCP), and a membrane-bound chl. a-chl. c2–peridinin- protein complex (acpPC), along with typical photosynthetic electron transport systems such as the photosystem II reaction centre and the chl. aP700 reaction centre complex of photosystem I (Iglesias-Prieto et al. 1991, 1993). Also associated with the thylakoids are the xanthophylls dinoxanthin, diadinoxanthin, diatoxanthin and the carotene, B-carotene. The pyrenoid contains the nuclear-encoded enzyme type II Ribulose-bis-phosphate- carboxylase-oxygenase (Rubisco) (Rowan et al. 1996), which is responsible for the catalysis of inorganic carbon dioxide into organic compounds.
All cultured isolates (i.e. strains) are capable of phenotypic adjustment in their capacity for light harvesting (i.e. photoacclimation), such as by altering the cellular Chl a and peridinin quota, as well as the size and number of photosynthetic units (Hennige et al., 2009). However, the ability to acclimate is a reflection of genetic differences among species that are differently adapted (evolved) to a particular photic environment (Iglesias-Prieto and Trench, 1994, 1997). For example, S. pilosum is characterized as a high light adapted species, while others are low light adapted (S. kawagutii) or adapted to a larger range in varying light fields (S. microadriaticum).

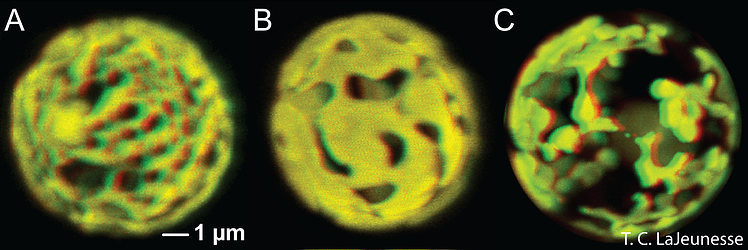
Figure 5. The reticulated chloroplast structures of (A) Symbiodinium goreaui (type C1), (B) “S. fitti” (type A3), and (C) “S. californium” (type E1) imaged in 3-D using confocal microscopy of chlorophyll autofluorescence (LaJeunesse et al. 2010a). © Todd LaJeunesse
The nucleus:
In general, the nucleus is centrally located and the nucleolus is often associated with the inner nuclear membrane. The chromosomes, as in other dinoflagellates, are seen as ‘permanently super-coiled’ DNA in transmission electron micrographs (TEM) (Blank & Trench 1985). The described species of Symbiodinium possess distinct chromosome numbers (ranging from 26-97; Trench & Blank 1987), which remain constant throughout all phases of the nuclear cycle. However, during M-phase, the volume of each chromosome is halved, as is the volume of each of the two resulting nuclei. Thus, the ratio of chromosome volume to nuclear volume remains constant. These observations are consistent with the interpretation that the algae are haploid, a conclusion supported by molecular genetic data (Santos & Coffroth 2003). During S-phase of the nuclear cycle the chromosomes do uncoil to facilitate DNA synthesis, and the volumes of both the chromosomes and the nucleus return to that seen in the G2 stage (Blank & Trench, 1985).
Other cytoplasmic organelles:
There are several additional organelles found in the cytoplasm of Symbiodinium. The most obvious of these is the structure referred to as the “accumulation body”. This is a membrane-bound vesicle (vacuole) with contents that are unrecognizable, but appear red or yellow under the light microscope. It may serve to accumulate cellular debris or act as an autophagic vacuole in which non-functional organelles are digested and their components recycled. During mitosis, only one daughter cell appears to acquire this structure. There are other vacuoles that may contain membranous inclusions (see Trench, 1974), while still others contain crystalline material variously interpreted as oxalate crystals or crystalline uric acid.
Life Cycle
The life cycle of Symbiodinium was first described from cells growing in culture media. For isolates that are in log phase growth, division rates occur every 1-3 days, with Symbiodinium cells alternating between a spherical, or coccoid, morphology and a smaller flagellated motile mastigote stage (see ‘Morphological Characteristics’). While several similar schemes are published that describe how each morphological state transitions to other, the most compelling life history reconstruction was deduced from light and electron microscopy and nuclear staining evidence (Fig. 6; Fitt and Trench 1983). During asexual propagation (sometimes referred to as mitotic or vegetative growth), cells undergo a diel cycle of karyokinesis (chromosome/nuclear division) in darkness. The mother cell then divides (cytokinesis) soon after exposure to light and releases two motile cells. The initiation and duration of motility varies among species (Fitt and Trench 1983). Approaching or at the end of the photoperiod the mastigotes cease swimming, release their flagella, and undergo a rapid metamorphosis into the coccoid form. As cultures reach stationary growth phase, fewer and fewer motile cells are observed, indicating slower division rates.
Large tetrads are occasionally observed, particularly when cells in stationary growth phase are transferred to fresh media. However, it is unknown whether this stage is the product two consecutive mitotic divisions, or perhaps a process that generates sexually competent motile cells (i.e. gametes), or is the end result of meiosis following gamete fusion. There is no cytological evidence for sexual recombination, and meiosis has never been observed, but population genetic evidence supports the view that Symbiodinium periodically undergo events of sexual recombination (Baillie et al. 2000; LaJeunesse 2001; Santos et al. 2004). How, when, and where, the sexual phase in their life history occurs remains unknown.

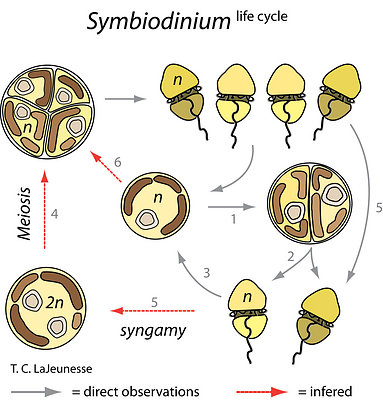
Figure 6. The Symbiodinium life cycle alternates between a motile mastigote phase and a coccoid phase (1 to 3). Rates of mitosis range from one to several days depending on nutrient availability and species. Two mastigotes are produced (2) as a result of mitosis. They emerge from the mother cell wall and remain swimming for several hours depending on the species, before transforming to the coccoid stage (3). Tetrads (the 4-cell stage) are produced and may either be the result of meiosis (4), the generation of isogamous gametes for sexual recombination (5), or from two successive rounds of mitosis during clonal propagation (6). Life cycle stages observed in culture are observed in hospite. Redrawn from Fitt and Trench (1983). © Todd LaJeunesse
Intracellular Symbionts
Symbiodinum are known primarily for their role as mutualistic endosymbionts. In hosts they usually occur in high densities, ranging from hundreds of thousands to millions per square centimeter (Fig. 7; Stimson et al. 2002). The successful culturing of swimming gymnodinioid cells from coral led to the discovery that “zooxanthellae” were actually dinoflagellates (Fig. 2A; Kawaguti 1944; McLaughlin & Zahl 1959). Each Symbiodinium cell is coccoid in hospite (living in a host cell) and surrounded by a membrane that originates from the host cell plasmalemma during phagocytosis (Figs 2B and 7). This membrane probably undergoes some modification to its protein content, which functions to limit or prevent phago-lysosome fusion (Fig. 2B; Colley & Trench 1985; Wakefield & Kempf 2001; Peng et al. 2010). The vacuole structure containing the symbiont is therefore termed the symbiosome. Under normal conditions, symbiont and host cells exchange organic and inorganic molecules that enable the growth and proliferation of both partners.

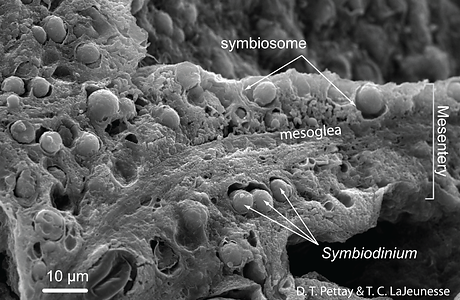
Figure 7. Symbiodinium reach high cell densities through prolific mitotic division in the endodermal tissues of many shallow tropical and sub-tropical cnidarians. This is a SEM of a freeze-fractured internal mesentery from a reef coral polyp (Porites porites) that shows the distribution and density of symbiont cells. © Todd LaJeunesse
Natural Services and Value
Symbiodinium are among the most studied of the Dinophyceae. Their mutualistic relationships with reef-building corals form the basis of a highly diverse and productive ecosystem. Coral reefs have economic benefits – valued at hundreds of billions of dollars each year – in the form of ornamental, subsistence, and commercial fisheries, tourism and recreation, coastal protection from storms, a source of new bioactive compounds for pharmaceutical development, and more (Moberg & Folke 1999). The economic value of Symbiodinium is thus immeasurable. Their continued productivity as symbionts and the functioning of tropical reef ecosystems are in serious jeopardy due to rapid ocean warming.
Symbiodinium and Coral Bleaching
The study of Symbiodinium biology is driven largely by a desire to understand global coral reef decline. A chief mechanism for widespread reef degradation has been stress-induced coral bleaching caused by anomalously high seawater temperature (Fig. 8). Bleaching is defined as the disassociation of the coral and the symbiont and/or loss of pigment within the alga, resulting in a precipitous loss of the animal’s brown pigmentation. Many Symbiodinium-cnidarian associations are negatively affected by sustained elevated temperature and high irradiance levels (including UVR) (Jokiel & Coles 1990; Lesser 1996; Fitt et al. 2001), extreme low temperatures (LaJeunesse et al. 2010c), low salinity (Goreau 1964), and other factors (Brown 2000). The bleached state is associated with decreased host calcification (Colombo-Pallotta et al. 2010), increased disease susceptibility (Brandt & McManus 2009) and, if prolonged, partial or total mortality (reviewed in Baker et al. 2008). The magnitude of mortality from a single bleaching event can be global in scale, and these episodes are predicted to become more common and severe as temperatures worldwide continue to rise (Hoegh-Guldberg et al. 2007). The physiology of a resident Symbiodinium species often regulates the bleaching susceptibility of a coral (Berkelmans & Van Oppen 2006; Sampayo et al. 2008). Therefore a significant amount of research has focused on characterizing the physiological basis of thermal tolerance (Robison & Warner, 2006; Warner et al. 2006; Ragni et al. 2008; Takahashi et al. 2008) and in identifying the ecology and distribution of thermally tolerant symbiont species (Lien et al. 2007; LaJeunesse et al. 2009; 2010b; Oliver & Palumbi 2009).

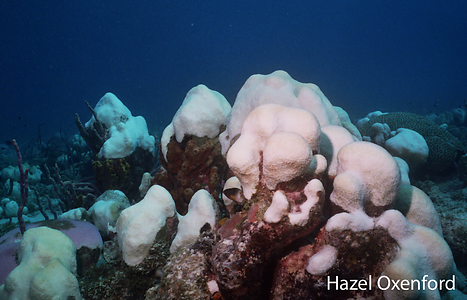
Figure 8. Coral bleaching involves the disassociation of coral-Symbiodinium symbioses in response to physiological stress brought on during periods of unusually high temperature and light. The presence of stress tolerant Symbiodinium spp. minimizes coral bleaching and mortality, and therefore ongoing research is interested in the physiology and host–specificity of these symbionts. This photo of “whitened” reef-building corals was taken off the coast of Barbados during the Fall 2005 Caribbean mass coral bleaching event. © Hazel Oxenford
Molecular Systematics of Symbiodinium
Preface
The advent of DNA sequence comparison initiated a rebirth in the ordering and naming of all organisms. The application of this methodology helped overturn the long held belief that Symbiodinium comprised a single species, a process which began in earnest with the morphological, physiological, and biochemical comparisons of cultured isolates. Now genetic markers are exclusively used to describe ecological patterns and deduce evolutionary relationships among morphologically cryptic members of this group. Foremost in the molecular systematics of Symbiodinium is to resolve ecologically relevant units of diversity (i.e. species).
Major phylogenetic disparity among Symbiodinium “Clades”
The earliest ribosomal gene sequence data indicated that Symbiodinium was comprised of lineages whose genetic divergence was similar to differences observed among dinoflagellates from different genera, families, and even orders (Rowan & Powers 1992). This large phylogenetic disparity among “clades” A, B, C, etc. was confirmed by analyses of the sequences of the mitochondrial gene coding for cytochrome oxidase 1 among Dinophyceae (Fig. 9; Stern et al. 2010). Most of these “clade” groupings comprise numerous reproductively isolated, genetically distinct lineages (see ‘Species diversity’), exhibiting different ecological and biogeographic distributions (see ‘Geographic distributions and patterns of diversity’). Given the over-simplified perceptions created by using only clade-level taxonomic designations for grouping Symbiodinium, future taxonomic revision of this genus is required. Many of these “clades” will probably be reclassified into distinct genera.

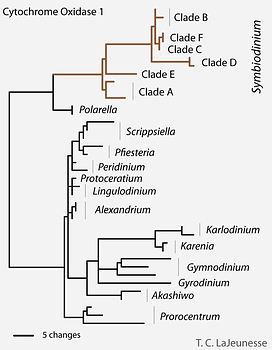
Figure 9. Genetic disparity between clades in the genus Symbiodinium compared to other dinoflagellates. Analysis of conserved mitochondrial sequences (CO1) and rDNA (SSU) suggest that a taxonomic revision of this group is required. © Todd LaJeunesse
Species diversity
The recognition of species diversity in this genus remained problematic for many decades due the challenges of identifying morphological and biochemical traits useful for diagnosing species (Trench & Blank 1987). Presently, phylogenetic, ecological, and population genetic data can be more rapidly acquired to resolve Symbiodinium into separate entities that are consistent with Biological, Evolutionary, and Ecological Species Concepts (Sampayo et al. 2009; LaJeunesse & Thornhill 2011). Most genetics-based measures of diversity have been estimated from the analysis of one genetic marker (e.g. LSU, ITS2, or cp23S), yet in recent studies these and other markers were analyzed in combination. The high concordance found among nuclear, mitochondrial and chloroplast DNA argues that a hierarchical phylogenetic scheme, combined with ecological and population genetic data, can unambiguously recognize and assign nomenclature to reproductively isolated lineages, i.e. species (Fig. 10).
The analysis of additional phylogenetic markers show that some Symbiodinium that were initially identified by slight differences in ITS sequences may comprise members of the same species (LaJeunesse & Thornhill 2011) whereas, in other cases, two or more genetically divergent lineages can possess the same ancestral ITS sequence (Santos et al. 2004; Finney et al. 2010). When analyzed in the context of the major species concepts (de Queiroz 2007), the majority of ITS2 sequence data provide a reasonable proxy for species diversity (sensu Thornhill et al. 2007; Sampayo et al. 2009; LaJeunesse & Thornhill 2011). Currently ITS2 types number in the hundreds and most communities of symbiotic cnidaria around the world still require comprehensive sampling. Furthermore, there appear to be a large number of unique species found in association with equally diverse species assemblages of soritid foraminifera (Pochon et al. 2007), as well as many other Symbiodinium that are exclusively free-living and found in varied, often benthic, habitats (Reimer et al. 2010) Given the potential species diversity of these ecologically cryptic Symbiodinium, the total species number may never be accurately assessed.

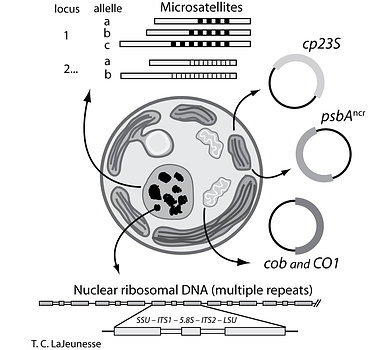
Figure 10. The investigation of Symbiodinium diversity, ecology, and evolution is enhanced by analysis of ribosomal and single copy nuclear, plastid, and mitochondrial DNA. The use of multiple markers, along with a hierarchical phylogenetic classification provides the genetic resolution necessary for investigating species diversity, biogeography, dispersal, natural selection, and adaptive radiations. (modified from Sampayo et al. 2009). © Todd LaJeunesse
Clone diversity and population genetics
Through the use of microsatellite markers, multilocus genotypes identifying a single clonal line of Symbiodinium can be resolved from samples of host tissue. It appears that most individual colonies harbor a single multilocus genotype (i.e. clone; Andras et al. 2011; Pettay et al. 2011). Extensive sampling within colonies confirms that many colonies harbor a homogeneous (clonal) Symbiodinium population. Additional genotypes do occur in some colonies, yet rarely more than two or three are found. When present in the same colony, multiple clones often exhibit narrow zones of overlap (Pettay et al. 2011). Colonies adjacent to each other on a reef may harbor identical clones, but across the host population the clone diversity of a particular Symbiodinium species is potentially large and comprises recombinant genotypes that are the product of sexual recombination. A clone tends to remain dominant in a colony over many months and years, but may be occasionally displaced or replaced. The few studies examining clone dispersal find that most genotypes have limited geographic distributions, but that dispersal and gene flow is likely influenced by host life history and mode of symbiont acquisition (e.g. horizontal vs. vertical).
Culturing Symbiodinium
Certain Symbiodinium strains and/or species are more easily cultured and can persist in artificial or supplemented seawater media (e.g. ASP–8A, F/2) for decades. The comparison of cultured isolates under identical conditions show clear differences in morphology, size, biochemistry, gene expression, swimming behavior, growth rates, etc. (e.g. Schoenberg and Trench 1980abc). This pioneering comparative approach initiated a slow paradigm shift in recognizing that this genus comprised more than a single species.
It should be noted that culturing is a selective process, and many Symbiodinium isolates growing on artificial media are not typical of the species normally associated with a particular host. Indeed most host–specific species have yet to be cultured. Samples for genetic analysis should be acquired from the source colony in order to match the resulting culture with the identity of the dominant and ecologically-relevant symbiont originally harbored by the animal (Santos et al. 2001; LaJeunesse 2002; Goulet & Coffroth 2003).
Symbiodinium Species Diversity, Ecology, and Biogeography
Geographic distributions and patterns of diversity
Symbiodinium are perhaps the best group for studying micro-eukaryote physiology and ecology for several reasons. 1) Phylogenetic and population genetic markers are now available that allow for detailed examination of their genetic diversity over broad spatial and temporal scales. 2) Large quantities Symbiodinium cells are readily obtained through the collection of hosts that harbor them. 3) Their association with animals provides an additional axis by which to compare and contrast ecological distributions.
The earliest genetic methods for assessing Symbiodinium diversity relied on low-resolution molecular markers that separated the genus into a few evolutionarily divergent lineages, referred to as “clades”. Previous characterizations of geographic distribution and dominance have focused on the clade-level of genetic resolution, but more detailed assessments of diversity at the species level are needed (Fig. 11). While members of a given clade may be ubiquitous, the species diversity within each clade is potentially large, with each species often having different ecological and geographic distributions related to their dispersal ability, host biogeography, and external environmental conditions. A small number of species occur in temperate environments where few symbiotic animals occur. As a result, these high latitude associations tend to be highly species specific.

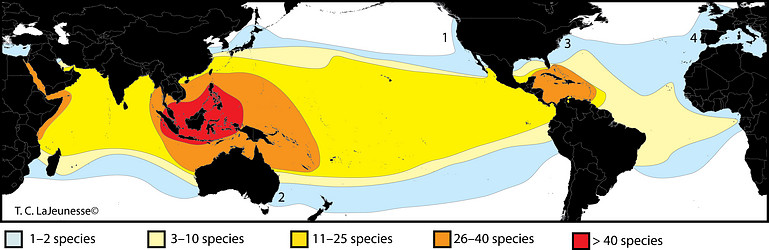
Figure 11. Global distribution and preliminary diversity estimate of common Symbiodinium species associated with cnidarians (rare “species” excluded). Internal transcribed spacer region 2 (ITS2) data (sensu LaJeunesse 2002) are used here as a proxy for species diversity. The diversity found in soritid foraminifera, the external environment, and other under-sampled habitats would increase these values potentially many fold. High latitudinal species are few (light blue) and are represented by 1) “Symbiodinium muscatinei” associated with anemones in the genus Anthopleura (LaJeunesse & Trench 2000); 2) A clade B type (B18/B18a) found in the coral Plesiastrea versipora from southeastern and southwestern Australia, respectively (Silverstein et al. 2011); 3) S. psygmophilum (type B2) associated with Astrangia poculata along the east coast of North America (Thornhill et al. 2008), and 4) type A’ harbored by cnidarians in the eastern North Atlantic and Mediterranean (Visram et al. 2006). © Todd LaJeunesse
Symbiodinium species diversity assigned to different ecological guilds
The large diversity of Symbiodinium revealed by genetic analyses is distributed non-randomly and appears to comprise several guilds with distinct ecological habits (Fig. 12). Of the many Symbiodinium characterized genetically, most are host-specific, mutualistic, and dominate their host (e.g. LaJeunesse 2002). Others may represent compatible symbionts that remain as low-abundance background populations because of competitive inferiority under the prevailing external environmental conditions (e.g. high light vs. low light; e.g. Rowan et al. 1997). Some may also comprise opportunistic species that may proliferate during periods of physiological stress and displace the normal resident symbiont and remain abundant in the host’s tissues for months to years before being replaced by the original symbiont (e.g. Toller et al. 2001; Thornhill et al. 2006; LaJeunesse et al. 2009). There are also those that rapidly infect and establish populations in host juveniles until being replaced by symbionts that normally associate with host adult colonies (e.g. Coffroth et al. 2001). Finally, there appears to be another group of Symbiodinium that are incapable of establishing endosymbiosis yet exist in environments around the animal or associate closely with other substrates (i.e. macro-algal surfaces, sediment surface; e.g. Porto et al. 2005; Reimer et al. 2010). Symbiodinium from functional groups 2, 3, and 4 are known to exist because they culture easily, however species with these life histories are difficult to study because of their low abundance in the environment (Fig. 12).

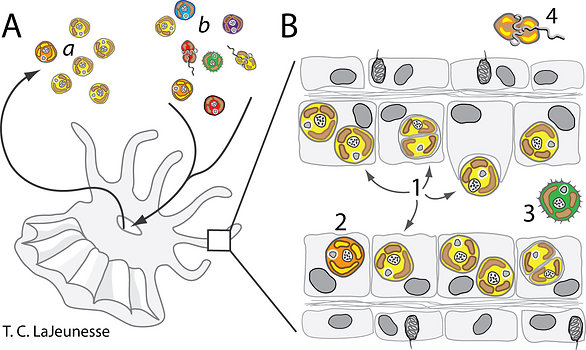
Figure 12. The distributions of Symbiodinium species comprising different ecological guilds in a coral reef ecosystem. A. Host cnidarians can expel millions of symbiont cells (viable and necrotic) per day into the surrounding environment (a). In turn these animals pass large volumes of water through their gastrovascular system for respiration and waste removal, a process that introduces numerous small particles including food and various other Symbiodinium spp. (indicated by differences in cell color) (b). B. The ecological niche, from the viewpoint of functional groups, differs among species of Symbiodinium. Several ecological guilds exist, including abundant host-specific and host-generalist species (1), low background and potentially opportunistic species (2), and non-symbiotic species closely associated with the coral’s biome (3) and/or occupying unrelated habitats (4). © Todd LaJeunesse
Free-living and “non-symbiotic” Symbiodinium
There are few examples of documented populations of free-living Symbiodinium (see references in Reimer et al. 2010). Given that most host larvae must initially acquire their symbionts from the environment, viable Symbiodinium cells occur outside the host. The motile phase is probably important in the external environment and facilitate the rapid infection of host larvae. The use of aposymbiotic host polyps deployed as "capture vessels" and the application of molecular techniques has allowed for the detection of environmental sources of Symbiodinium (Coffroth et al. 2006; Thornhill et al. 2006). With these methods employed, investigators may resolve the distribution of different species on various benthic surfaces (e.g. Porto et al. 2005) and cell densities suspended in the water column (e.g. Manning & Gates 2008). The genetic identities of cells cultured from the environment are often dissimilar to those found in hosts. These likely do not form endosymbioses and are entirely free-living; they are different from “dispersing” symbiotic species (LaJeunesse 2002). Learning more about the "private lives" of these environmental populations and their ecological function will further our knowledge about the diversity, dispersal success, and evolution among members within this large genus.
References
Andras JP, Kirk NL, Harvell CW (2011) Range-wide population genetic structure of Symbiodinium associated with the Caribbean sea fan coral, Gorgonia ventalina. Mol Ecol 20:2525-2542
Baillie BK, Belda-Baillie CA, Silvestre V, Sison M, Gomez AV, Gomez ED, Monje V (2000). Genetic variation in Symbiodinium isolates from giant clams based on random-amplified-polymorphic DNA (RAPD) patterns. Mar Bio 136:829-36
Baker AC, Glynn PW, Riegl B (2008) Climate change and coral reef bleaching: an ecological assessment of long-term impacts, recovery trends and future outlook. Estuar Coast Shelf S 80:435-471
Banaszak AT, Iglesias-Prieto R, Trench RK (1993) Scrippsiella velellae sp. nov. (Peridiniales) and Gloeodinium viscum sp. nov. (Phytodiniales), dinoflagellate symbionts of two hydrozoans (Cnidaria). J Phycol 29:517-28
Berkelmanns, R. & van Oppen, M.J.H. 2006 Flexible partners in coral symbiosis: a 'nugget of hope' for coral reefs in an era of climate change. Proc. R. Soc. Lond. B Biol. Sci. 273, 2305-2312.
Blank RJ, Trench RK (1985) Speciation and symbiotic dinoflagellates. Science 229:656-658
Blank RJ, Trench RK (1986) Nomenclature of endosymbiotic dinoflagellates. Taxon 35:286-94
Blank RJ, Huss VAR (1989) DNA divergency and speciation in Symbiodinium (Dinophyceae). P. Syst Evo. 163:153-63
Brandt ME, McManus JW (2009) Disease incidence is related to bleaching extent in reef-building corals. Ecology 90:2859-2867
Brown BE (2000) The significance of pollution in eliciting the ‘bleaching’ response in symbiotic cnidarians. Int J Environ Pollut 13:392-415
Coffroth MA, Santos SR, Goulet TL (2001) Early ontogenic expression of specificity in a cnidarian-algal symbiosis. Mar Ecol Prog Ser 222:85-96
Coffroth MA, Lewis CF, Santos SR (2006) Environmental populations of symbiotic dinoflagellates in the genus Symbiodinium can initiate symbioses with reef cnidarians. Curr Biol 16:987-987
Colley NJ, Trench RK (1983) Selectivity in phagocytosis and persistence of symbiotic algae by the scyphistoma stage of the jellyfish Cassiopeia xamachana. Proc R Soc Lond B 219: 61-82
Colombio-Pallotta MF, Rodriguez-Roman A, Iglesias-Prieto R (2010) Calcification in bleached and unbleached Montastrea faveolata: evaluating the role of oxygen and glycerol. Coral Reefs 29:899-907
Diaz-Almeyda E, Thome PE, Hafidi M, Iglesias-Prieto R (2010) Differential stability of photosynthetis membranes and fatty acid composition at elevated temperature in Symbiodinium. Coral Reefs 30:217-225
Dodge JD, Greuet C (1987) Dinoflagellate ultrastructure and complex organelles. In Botanical Monographs, vol. 21, the biology of dinoflagellates (eds. F. J. R. Taylor), Blackwell Scientific Publications, Oxford, pp. 92-142
Fitt WK, Trench RK (1983) The relation of diel patterns of cell division to diel patterns of motility in the symbiotic dinoflagellate Symbiodinium microadriaticum Freudenthal in culture. New Phytol 94:421-432
Fitt WK, Brown BE, Warner ME, Dunne RP (2001) Coral bleaching: interpretation of thermal tolerance limits and thermal thresholds in tropical corals. Coral Reefs 20:51-56
Freudenthal HD (1962) Symbiodinium gen. nov. and Symbiodinium microadriaticum sp. nov., a zooxanthella: Taxonomy, life cycle and morphology. J Protozool 9:45-52
Goreau TF (1964) Mass expulsion of Zooxanthellae from Jamaican reef communties after Hurricane Flora. Science 145:383-386.
Goulet TL, Coffroth MA (2003) Genetic composition of zooxanthellae between and within colonies of the octocoral Plexaura kuna, based on small subunit rDNA and multilocus DNA fingerprinting. Mar Biol 142:233-239
Hansen G, Daugbjerg N (2009) Symbiodinium natans sp. nov.: a “free-living dinoflagellate from Tenerife (Northeast-Atlantic Ocean). J. Phycol. 45:251-63.
Hoegh-Guldberg O, Mumby PJ, Hooten AJ, Steneck RS, Greenfield P, Gomez E, Harvell CD, Sale PF, Edwards AJ, Caldeira K, Knowlton N, Eakin EM, Iglesias-Prieto R, Muthiga N, Bradbury RH, Dubi A, Hatziolos ME (2007) Coral reefs under rapid climate change and ocean acidification. Science 318:1737-1742
Iglesias-Prieto R, Govind NS, Trench RK (1991) Apoprotein Composition and spectroscopic characterization of the water-soluble peridinin—chlorophyll a—proteins from three symbiotic dinoflagellates. Proc Roy Soc B 246:275-283
Iglesias-Prieto R, Govind NS, Trench RK (1993) Isolation and characterization of three membrane-bound chlorophyll-protein complexes from four dinoflagellate species. Proc Roy Soc B 1294:381-392
Jokiel PL, Coles SL (1990) Response of Hawaiian and other Indo-Pacific reef corals to elevated temperature. Coral Reefs 8:155-162
Jones RJ, Yellowlees D (1997) Regulation and control of intracellular algae (= zooxanthllae) in hard corals. Phil Trans R Soc Lond B 352 : 457-468
Kawaguti S (1944) On the physiology of reef corals. VII. Zooxanthellae of the reef corals is Gymnodinium sp. Dinoflagellata; its culture in vitro. Palau Tropical Biological Station Studies 2:265-275
LaJeunesse TC, Trench RK (2000) Biogeography of two species of Symbiodinium (Freudenthal) inhabiting the intertidal sea anemone Anthopleura elegantissimi (Brandt). Biol Bull 199:126-134
LaJeunesse TC (2001) Investigating the biodiversity, ecology, and phylogeny of endosymbiotic dinoflagellates in the genus Symbiodinium using the ITS region: In search of a “species” level marker. J Phyco. 37:866-80
LaJeunesse TC (2002) Diversity and community structure of symbiotic dinoflagellates from Caribbean coral reefs. Mar Biol 141:387-400
LaJeunesse TC, Lambert G, Andersen RA, Coffroth MA, Galbraith DW (2005) Symbiodinium (Pyrrhophyta) genome sizes (DNA content) are smallest among dinoflagellates. J. Phycol. 41:880-6.
LaJeunesse TC, Finney JC, Smith R, Oxenford H (2009) Outbreak and persistence of opportunistic symbiotic dinoflagellates during the 2005 Caribbean mass coral ‘bleaching’ event. Proc. Roy Soc Lond, B 276: 4139-4148.
LaJeunesse TC, Fitt WK, Schmidt GW (2010a) The reticulated chloroplasts of zooxanthellae (Symbiodinium) and differences in chlorophyll localization among life cycle stages. Coral Reefs 29: 627
LaJeunesse TC, Pettay T, Sampayo EM, Phongsuwan N, Brown B, Obura D, Hoegh-Guldberg O, Fitt WK (2010b) Special Paper: Long-standing environmental conditions, geographic isolation and host–symbiont specificity influence the relative ecological dominance and genetic diversification of coral endosymbionts in the genus Symbiodinium. J Biogeography 37: 785-800.
LaJeunesse TC, Smith R, Walther M, Pettay T, McGinley M, Aschaffenburg M, Medina-Rosas P, Cupul-Magana AL, Perez A L, Reyes-Bonilla H, Warner ME (2010c) Host-symbiont recombination vs. natural selection in the response of coral-dinoflagellate symbioses to environmental disturbance. Proc Royal Soc B 277: 2925-2934
LaJeunesse TC, Thornhill DJ (2011) Improved resolution of reef-coral endosymbiont (Symbiodinium) species diversity, ecology, and evolution through psbA non-coding region genotyping. PlosOne e29013
Lee RE (2008) Phycology, 4th edition. Cambridge University Press. New York. 547pp.
Lesser MP (1996) Elevated temperatures and ultraviolet radiation cause oxidative stress and inhibit photosynthesis in symbiotic dinoflagellates. Limnol Oceanogr 41:271-283
Lien YT, Nakano Y, Plathong S, Fukami H, Wang JT. Chen CA (2007) Occurrence of the putatively heat-tolerant Symbiodinium phylotype D in high-latitudinal marginal coral communities. Coral Reefs, 26: 35-44.
Manning MM, Gates RD (2008) Diversity in populations of free-living Symbiodinium from a Caribbean and Pacific reef. Limno. Oceanogr 53:1853-1861
Markell DA, Trench RK, Iglesias-Prieto R (1992) Macromolecules associated with the cell-walls of symbiotic dinoflagellates. Symbiosis 12:19-31
McLaughlin JJA, Zahl PA (1959) Axenic zooxanthellae from various invertebrate hosts. Ann NY Acad S 77:55-72
Moberg F, Folke C (1999) Ecological goods and services of coral reef ecosystems. Ecol Econ 29:215-233
Peng S, Wang Y, Wang L, Chen WU, Lu C, Fang L, Chen C (2010) Proteomic analysis of symbiosome membranes in Cnidaria-dinoflagellate endosymbiosis. Proteomics 10:1002-1016
Pettay DT, Wham DC, Pinzón JH, LaJeunesse TC (2011) Genotypic diversity and spatial¬–temporal distribution of Symbiodinium clones in an abundant reef coral. Mol Ecol 20: 5197-5212
Pochon X, Garcia-Cuestos L, Baker AC, Castella E, Pawlowski J (2007) One-year survey of a single Micronesian reef reveals extraordinarily rich diversity of Symbiodinium types in sorited foraminifera. Coral Reefs 26:867-82
Porto I, Granados C, Restrepo JC, Sanchez JA (2008) Macroalgal-associated dinoflagellates belonging to the genus Symbiodinium in Caribbean reefs. PLoS ONE 3:e2160
de Queiroz K (2007) Species concepts and species delimitation. Syst Bio. 56:879–86
Ragni M, Airs R, Hennige S, Suggett D, Warner M, Geider R, (2010) PSII photoinhibition and photorepair in Symbiodinium (Pyrrhophyta) differs between thermally tolerant and sensitive phylotypes. Mar Ecol Prog Ser 406: 57-70
Reimer JD, Shah MMR, Sinniger F, Yanagi K, Suda S (2010) Preliminary analyses of cultured Symbiodinium isolated from sand in the oceanic Ogasawara Islands, Japan. Mar Biodiv 40:237-247
Robison, JR , Warner ME (2006) Differential impacts of photoacclimation and thermal stress on the photobiology of four different phylotypes of Symbiodinium (Pyrrhophyta). J Phycol 42:568-579
Rowan, R. 2004 Thermal adaptation in reef coral symbionts. Nature 430, 742.
Rowan R, Powers DA (1992) Ribosomal-RNA sequences and the diversity of symbiotic dinoflagellates (zooxanthellae). Proc Natl Acad Sci USA 89:3639-3643
Rowan R & Powers DA (1991) A molecular genetic classification of zooxanthellae and the evolution of animal-algal symbiosis. Science 251:1348-51.
Rowan R, Whitney SM, Fowler A, Yellowlees D (1996) Rubisco in marine symbiotic dinoflagellates: Form II enzymes in eukaryotic oxygenic phototrophs encoded by a nuclear multigene family. Plant Cell 8:539-553.
Rowan, R., Knowlton, N., Baker, A. & Jara, J. 1997 Landscape ecology of algal symbionts creates variation in episodes of coral bleaching. Nature 388, 265–269.
Sampayo EM, Ridgeway T, Bongaerts P. Hoegh-Gulberg O. (2008) Bleaching susceptibility and mortality of corals are determined by fine-scale differences in symbiont type. Proc. Natl Acad. Sci. 105, 10 444–10 449. (doi:10.1073/pnas.0708049105)
Sampayo E, Dove S, LaJeunesse TC (2009) Cohesive molecular genetic data delineate species diversity in the dinoflagellate genus Symbiodinium. Mol Eco. 18:500-519
Santos SR, Taylor DJ, Coffroth MA (2001) Genetic comparisons of freshly isolated vs. cultured symbiotic dinoflagellates: implications for extrapolating to the intact symbiosis. J Phycol 37:900-12
Santos SR, Coffroth MA (2003) Molecular genetic evidence that dinoflagellates belonging to the genus Symbiodinium Freudenthal are haploid. Biol Bull 204:10-20
Santos SR, Shearer TL, Hannes AR, Coffroth MA (2004) Fine scale diversity and specificity in the most prevalent lineage of symbiotic dinoflagellates (Symbiodinium, Dinophyta) of the Caribbean. Mo. Ecol 13: 459-469
Schoenberg DA, Trench RK (1980a) Genetic variation in Symbiodinium (=Gymnodinium) microadriaticum Freudenthal, and specificity in its symbiosis with marine invertebrates. I. Isoenzyme and soluble protein patterns of axenic cultures of S. microadriaticum. Proc R Soc Lond B 207:405-27
Schoenberg DA, Trench RK (1980b) Genetic variation in Symbiodinium (=Gymnodinium) microadriaticum Freudenthal, and specificity in its symbiosis with marine invertebrates. II. Morphological variation in S. microadriaticum. Proc R Soc Lond B 207:429-44
Schoenberg DA, Trench RK (1980c) Genetic variation in Symbiodinium (=Gymnodinium) microadriaticum Freudenthal, and specificity in its symbiosis with marine invertebrates. III. Specificity and infectivity of S. microadriaticum. Proc R Soc Lond B 207:445-60
Silverstein RN, Correa AMS, LaJeunesse TC, Baker AC (2011) Novel algal symbiont (Symbiodinium spp.) diversity in reef coral of Western Australia. Mar Ecol Prog Ser 422:63-75
Stern RF, Horak A, Andrew RL, Coffroth MA, Andersen RA, Kupper FC, Jameson I, Hoppenrath M, Veron B, Kasai F, Brand J, James ER, Keeling PJ (2010) Environmental barcoding reveals massive dinoflagellate diversity on marine environments. PLoS ONE 5:e13991
Stimson J, Sakai K, Sembali H (2002) Interspecific comparison of the symbiotic relationship in corals with high and low rates of bleaching-induces mortality. Coral Reefs 21:409-421
Takahashi S, Whitney S, Itoh S, Maruyama T, Badger M (2008) Heat stress causes inhibition of de novo synthesis of antenna proteins and photobleaching in cultured Symbiodinium. Proc Nat Acad Sci 105:4203-4208
Taylor FJR (1987) Dinoflagellate morphology. In Botanical Monographs, vol. 21, The Biology of Dinoflagellates (eds. F. J. R. Taylor) Oxford: Blackwell Scientific Publications, pp. 24-91.
Thornhill DJ, LaJeunesse TC, Kemp DW, Fitt WK, Schmidt GW (2006) Multi-year, seasonal genotypic surveys of coral-algal symbioses reveal prevalent stability or post-bleaching reversion. Mar Biol 148:711-722
Thornhill DJ, LaJeunesse TC, Santos SR (2007) Measuring rDNA diversity in eukaryotic microbial systems: how intragenomic variation, pseudogenes, and PCR artifacts confound biodiversity estimates. Mol Ecol 24:5326-5340
Toller WW, Rowan R, Knowlton N (2001) Repopulation of zooxanthellae in the Caribbean corals Montastraea annularis and M. faveolata following experimental and disease-associated bleaching. Biol. Bull. 201:360-373
Trench RK (1974) Nutritional potentials in Zoanthus sociathus (Coelenterata, Anthozoa). Helgol Wiss Meeresunters 26:174-216
Trench RK, Colley NJ, Fitt WK (1981) Recognition phenomena in symbioses between marine invertebrates and "zooxanthellae"; uptake, sequestration and persistence. Ber. Deutsch. Bot. Ges.Bd. 94, S. 529-545
Trench RK, Blank RJ (1987) Symbiodinium microadriaticum Freudenthal, S. goreauii sp. nov., S. kawagutii sp. nov. and S. pilosum sp. nov.: Gymnodinioid dinoflagellate symbionts of marine invertebrates. J Phycol 23:469-481
Trench RK, Winsor H (1987) Symbiosis with dinoflagellates in two pelagic flatworms, Amphiscolops sp. and Haplodiscus sp. Symbiosis 3:1-21
Udy JW, Hinde R, Vesk M (1993) Chromosomes and DNA in Symbiodinium from Australian hosts. J Phycol. 29:314-20
Visram S, Wiedenmann, Douglas AE (2006) Molecular diversity of symbiotic algae of the genus Symbiodinium (Zooxanthellae) in cnidarians of the Mediterranean Sea. J. Mar Biol Ass. UK 86:1281-1283.
Wakefield TS, Farmer MA, Kempf SC (2000) Revised description of the fine structure of in situ “zooxanthellae” genus Symbiodinium. Biol Bull 199:76-84
Wakefield TS, Kempf SC (2001) Development of host- and symbiont-specific monoclonal antibodies and confirmation of the origin of the symbiosome membrane in a Cnidarian-Dinoflagellate symbiosis. Biol Bull 200:127-143
Warner ME, LaJeunesse TC, Robison JD, Thur RM (2006) The ecological distribution and comparative photobiology of symbiotic dinoflagellates from reef corals in Belize: potential implications for coral bleaching. Limnol. Oceanogr. 51:1887-1897.
Wilkinson C (2004) Status of coral reefs of the world: 2004, vol. 2, Australian Institute of Marine Science, Townsville, pp. 303-547
Information on the Internet
- Symbiosis Ecology and Evolution Laboratory, Penn State
- Scott R. Santos Laboratory, Auburn University
- Buffalo University Reef Research, New York University, Buffalo
- Geosymbio, University of Hawaii
- NOAA Coral Reef Conservation Program: Symbiotic Algae
- AIMS: Microbial Partnerships
- AIMS: Coral Bleaching
About This Page
Acknowledged ContributorsMary Alice Coffroth, Dustin Kemp, James D. Reimer, Scott R. Santos, Daniel J. Thornhill, Mark E. Warner.
Todd LaJeunesse
The Pennsylvania State University
Robert K. Trench
Department of Biological Sciences and Marine Science Institute, University of California, Santa Barbara
Correspondence regarding this page should be directed to Todd LaJeunesse at , John E. Parkinson at , and Robert K. Trench at
Page copyright © 2012 Todd LaJeunesse, , and Robert K. Trench
Page: Tree of Life
Symbiodinium.
Authored by
Todd LaJeunesse, John E. Parkinson, and Robert K. Trench.
The TEXT of this page is licensed under the
Creative Commons Attribution-NonCommercial License - Version 3.0. Note that images and other media
featured on this page are each governed by their own license, and they may or may not be available
for reuse. Click on an image or a media link to access the media data window, which provides the
relevant licensing information. For the general terms and conditions of ToL material reuse and
redistribution, please see the Tree of Life Copyright
Policies.
- First online 04 July 2012
- Content changed 04 July 2012
Citing this page:
LaJeunesse, Todd, John E. Parkinson, and Robert K. Trench. 2012. Symbiodinium. Version 04 July 2012. http://tolweb.org/Symbiodinium/126705/2012.07.04 in The Tree of Life Web Project, http://tolweb.org/