The focus of this treehouse will be upon two of the most studied species in the phylum Deinococcus-Thermus: Thermus aquaticus and Deinococcus radiodurans. The first section of this treehouse will investigate the environmental origins of T. aquaticus. The climatic events in its environment and the stresses associated with it are crucial in shaping various aspects of the organism. I will discuss an evolutionary adaptation required to deal with the effects of thermal stressors on T. aquaticus. It was the founder of evolution, Charles Darwin himself, who was one of the first to conclude that biodiversity stems from the wide range of microclimates available by stating that “a change in the conditions of life, by specially acting on the reproductive system, causes or increases variability.”1 Such a distinguished bacterial phylum as Deinococcus-Thermus is uniquely capable of many biological feats. We will investigate a second adaptation in the family of organisms alternatively known as “The Bacteria from Hades”. The second section of the treehouse will focus on the species D. radiodurans which is particularly well known for its ability to withstand high doses of ionizing radiation, ultraviolet radiation, high levels of reactive oxygen species and extreme xeric conditions. It is the ability to withstand ionizing radiation that will be discussed.
Thermus aquaticus
Thermus aquaticus is a bacterium that has risen to fame thanks to the widespread use of its DNA polymerase in the polymerase chain reaction technique (PCR). T. aquaticus is a thermophile. This means that it proliferates in hot environments.2 To survive in a tough environment such as the geothermal springs of Yellowstone National Park, where it was first discovered,3 T. aquaticus requires a set of proteins that can survive the geothermal springs too.

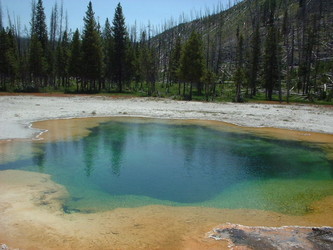
Emerald Pool, a hot spring in Yellowstone National Park, the habitat for Thermus aquaticus. Aesthetically pleasing, but not the type of neighbourhood you'd want to grow up in. Much too hot. © 2003 Angela & Adam.
DNA polymerase (DNApol) is an enzyme involved in the replication of DNA by contributing to synthesis of the daughter strand. In order to synthesize an additional copy of its DNA, T. aquaticus’s DNApol (Taq pol) is stable at elevated temperatures. The maximum formation of DNA filaments using Taq pol is achieved at the optimal temperature of 70 °C 4 and it can synthesize filaments up to temperatures of 97.5 °C.2 Stability at this increased temperature is a biological achievement because the typical proteins in most eukaryotes and Eubacteria such as Escherichia coli are stable around 37 °C. As a protein’s conformation contributes directly to its function, increased temperature outside the optimal range causes the denaturation of proteins. Denaturation is a process where proteins lose their conformation and ability to function. The increased stability of T. aquaticus’s proteins is thought to be achieved through increased non-covalent ionic interactions within the protein.2
Elevated temperatures, such as those encountered above 55 °C are considered to be extreme and suitable only for thermophilic organisms. This is because typical temperatures at the surface of the Earth do not exceed 50 °C. 2 For proof of how stressful excess heat can be, spend 15 minutes in a sauna. Your body can adapt in the short term by sweating to deal with the stress of heat. This allows a human body to maintain its homeostatic temperature of 37°C. T. aquaticus has adapted to high temperatures so that it is actually quite comfortable at temperatures near that of boiling water. One possible source of these adaptations is spontaneous mutations that allowed it to invade the geothermal niche. However, a newly formed theory is that stress actually induces mutations.5 Induced mutations can allow adaptations to the stress. Though this hypothesis has not been tested, the evolution of a heat-tolerant enzyme may have been induced by the bacteria’s origins in hot springs worldwide.
The adaptations of extremophiles (organisms that survive under extreme conditions) such as T. aquaticus may prove to be particularly useful in deciphering the origins of the Tree of Life. Environments such as the geothermal springs are hypothesized to reflect conditions similar to those of the early Earth where life arose. 6
Deinococcus radiodurans
Deinococcus radiodurans has encountered severe scrutiny of its abilities from scientific research. It was discovered in 1956 in a piece of meat following γ radiation intended to sterilize the meat. It has the ability to withstand acute ionizing radiation (IR) of 10 kGy without undergoing cell death. 7 That is 5 times the lethal dosage of radiation for E. coli, and 2,000 times the dosage that is lethal to humans.7 There are several adaptations that D. radiodurans has that enable it to withstand such high levels of radiation. These include DNA repair, protein protection, DNA damage export, genetic redundancy and desiccation recovery.8

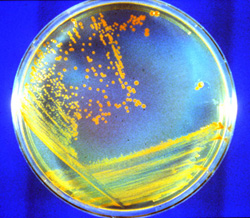
Deinococcus radiodurans © Scott N. Montross
D. radiodurans has several unique mechanisms utile in DNA repair. Some of these are known and others remain orphans, with yet-to-be-determined functions. Recent research has shown that some of D. radiodurans's proteins are specific to the species, such as DdrA. 9 This protein provides stability to DNA ends and eases repair of DNA following radiation. It is similar to the eukaryotic protein Rad52 which binds to 3’ single-stranded DNA during recombination. Homologous recombination is the main pathway for DNA repair in D. radiodurans with the non-homologous end joining pathway virtually absent.10 The results of tests involving wild type and a DdrA-deletion yielded a drop in survival from approximately 10% to 0.1% following 20 kGy radiation. Thus, it has been shown that DdrA contributes to the extreme radiotolerance of D. radiodurans.9
The efficacy of DNA repair is increased by the protection afforded by Manganese accumulation in D. radiodurans. It was first observed that extreme levels of IR resistance such as those of D. radiodurans were correlated with high intracellular Mn(II) concentrations. When Mn accumulation and redox cycling were inhibited, D. radiodurans became sensitive to radiation.10 A key difference between radiation-sensitive bacteria and radiation-resistant bacteria is their susceptibility to protein oxidation.10 Radiation-sensitive bacteria are highly susceptible to protein oxidation, whereas high levels of intracellular Mn in radiation-resistant bacteria provide them with a lessened susceptibility to protein oxidation. Mn complexes help D. radiodurans recover from oxidative damage to proteins by preventing Fe-induced carbonylation.10 Oxidative protein damage has the potential to disrupt cellular pathways that lead to DNA repair. Also, damaged proteins can promote mutagenesis via misrepairs. 11 Comparisons of the genome of D. radiodurans and Deinococcus geothermalis contribute to the hypothesis that D. radiodurans has a largely conventional set of DNA repair proteins that are functional in situations where IR-sensitive bacteria lose the ability to repair DNA. However, there are several genes that confer resistance to IR, that are specific to the Deinococcus family, which are indicative of novel resistance mechanisms.10
Once DNA damage has been incurred by radiation, D. radiodurans degrades the damaged DNA and exports it to the cytoplasm and outside the cell. The degraded DNA takes the form of approximately 2kb oligonucleotides. The export of DNA damage is hypothesized to have two functions11:
- To prevent reincorporation of damaged and mutated DNA sequences via DNA repair mechanisms such as recombination.
- Export of damaged DNA is an important part of signal transduction that initiates DNA repair mechanisms.
Genetic redundancy contributes to D. radiodurans's ability to survive DNA damage. Each cell contains 4 to 10 copies of its genome. Polyploidy can provide additional sources of backup DNA information in cases of chronic and accumulating DNA damage. Similar to the usefulness of a template strand in DSBR, the extra copies can ensure that at least one full copy of the genome survives radiation. 7 The structure of the genomes facilitates the survival and reformation of DNA, as they appear stacked upon each other.
D. radiodurans's ability to withstand high levels of DNA damage incurred by radiation may also be linked to its ability to withstand desiccation. 10 The bacterial champion of desiccation, D. radiodurans has been known to survive 6 years in a desiccator. A niche that has been exploited multiple times by D. radiodurans is one that is xeric and has low levels of nutrients. They have been found in this type of environment all over the world which indicates that they have evolved under prolonged environmental stress. It has been shown that extended xeric conditions induce DNA damage.10 Efficient DNA repair is one of the evolved adaptations to xeric stress, and it has been shown that strains of IR-sensitive D. radiodurans are also sensitive to xeric stress.
Conclusion
While the phylum Deinococcus-Thermus is widely recognized as one of the most extremophilic clades of organisms, within the phylum there is a standard for the achievement of extraordinary biological adaptations. Being able to withstand radiation, high temperatures and desiccators are all part of a day’s work for these microbes. This leaves me wondering, where will we find them next?
Information on the Internet
- Deinococcus radiodurans - a radiation-resistant bacterium
- Bacteria Genomes - DEINOCOCCUS RADIODURANS European Bioinformatics Institute